To view the full content, login to your account,
or start your 30-day FREE Trial today.
FREE TRIAL
LOGIN
Forgot Password?
Explore some of our unlocked OE Originals below!
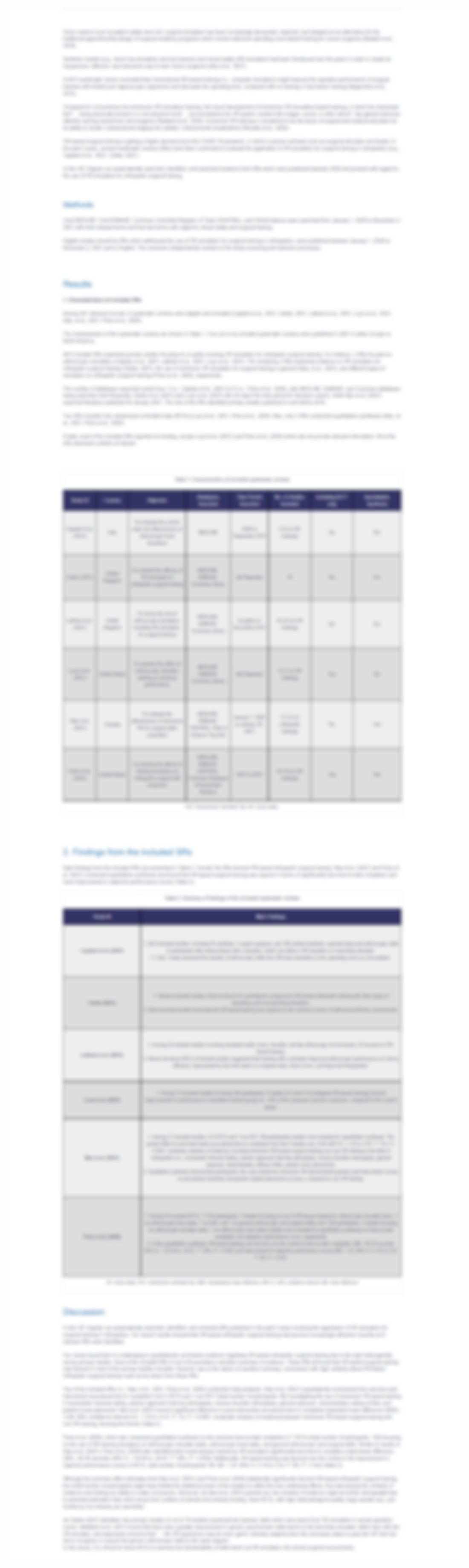
Join the Conversation
Please Login or Join to leave comments.